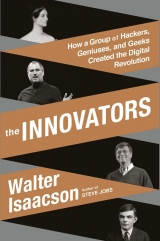
Текст книги "The Innovators: How a Group of Inventors, Hackers, Geniuses, and Geeks Created the Digital Revolution"
Автор книги: Walter Isaacson
Жанр:
Биографии и мемуары
сообщить о нарушении
Текущая страница: 6 (всего у книги 42 страниц)
Howard Aiken (1900–1973) at Harvard in 1945.
John Mauchly (1907–80) circa 1945.
J. Presper Eckert (1919–95) circa 1945.
Eckert (touching machine), Mauchly (by pillar), Jean Jennings (in back), and Herman Goldstine (by Jennings) with ENIAC in 1946.
Either way, the letter upset Atanasoff, who had still not succeeded in prodding his lawyer into filing any patent claims. He responded to Mauchly rather brusquely within a few days: “Our attorney has emphasized the need of being careful about the dissemination of information about our device until a patent application is filed. This should not require too long, and, of course, I have no qualms about having informed you about our device, but it does require that we refrain from making public any details for the time being.”60 Amazingly, this exchange still did not provoke Atanasoff or the lawyer to make a filing for patents.
Mauchly proceeded to forge ahead during that fall of 1941 with his own design for a computer, which he correctly believed drew ideas from a wide variety of sources and was very different from what Atanasoff had built. In his summer course, he met the right partner to join him in the endeavor: a graduate student with a perfectionist’s passion for precision engineering, who knew so much about electronics that he served as Mauchly’s lab instructor, even though he was twelve years younger (at twenty-two) and didn’t yet have his PhD.
J. PRESPER ECKERT
John Adam Presper Eckert Jr., known formally as J. Presper Eckert and informally as Pres, was the only child of a millionaire real estate developer in Philadelphia.61 One of his great-grandfathers, Thomas Mills, invented the machines that made salt water taffy in Atlantic City and, as important, created a business to manufacture and sell them. As a young boy, Eckert was driven by his family’s chauffeur to the William Penn private school, founded in 1689. But his success came not from the privileges of birth but from his own talents. He won a citywide science fair at age twelve by building a guidance system for model boats using magnets and rheostats, and at fourteen he devised an innovative way to use household current to eliminate troublesome batteries for the intercom system in one of his father’s buildings.62
In high school Eckert dazzled his classmates with his inventions, and he made money by building radios, amplifiers, and sound systems. Philadelphia, the city of Benjamin Franklin, was then a great electronics center, and Eckert spent time at the research lab of Philo Farnsworth, one of the inventors of television. Although he was accepted by MIT and wanted to go there, his parents did not wish him to leave. Pretending to have suffered financial setbacks because of the Depression, they pressured him to go to Penn and live at home. He did rebel, however, against their desire that he study business; instead he enrolled in the university’s Moore School of Electrical Engineering because he found the subject more interesting.
Eckert’s social triumph at Penn was creating what he called an “Osculometer” (from the Latin word for mouth), which purported to measure the passion and romantic electricity of a kiss. A couple would hold the handles of the device and then kiss, their lip contact completing an electric circuit. A row of bulbs would light up, the goal being to kiss passionately enough to light up all ten and set off a blast from a foghorn. Smart contestants knew that wet kisses and sweaty palms increased the circuit’s conductivity.63 Eckert also invented a device that used a light-modulating method to record sound on film, for which he successfully applied for a patent at age twenty-one, while still an undergraduate.64
Pres Eckert had his quirks. Filled with nervous energy, he would pace the room, bite his nails, leap around, and occasionally stand atop a desk when he was thinking. He wore a watch chain that wasn’t connected to a watch, and he would twirl it in his hands as if it were rosary beads. He had a quick temper that would flare and then dissolve into charm. His demand for perfection came from his father, who would walk around construction sites carrying a large pack of crayons with which to scrawl instructions, using different colors to indicate which worker was responsible. “He was sort of a perfectionist and made sure you did it right,” his son said. “But he had a lot of charm, really. He got things done most of the time by people wanting to do the stuff.” An engineer’s engineer, Eckert felt that people like himself were necessary complements to physicists such as Mauchly. “A physicist is one who’s concerned with the truth,” he later said. “An engineer is one who’s concerned with getting the job done.”65
ENIAC
War mobilizes science. Over the centuries, ever since the ancient Greeks built a catapult and Leonardo da Vinci served as the military engineer for Cesare Borgia, martial needs have propelled advances in technology, and this was especially true in the mid-twentieth century. Many of the paramount technological feats of that era—computers, atomic power, radar, and the Internet—were spawned by the military.
America’s entry into World War II in December 1941 provided the impetus to fund the machine that Mauchly and Eckert were devising. The University of Pennsylvania and the Army’s Ordnance Department at Aberdeen Proving Ground had been tasked with producing the booklets of firing-angle settings needed for the artillery being shipped to Europe. In order to be aimed properly, the guns required tables that factored in hundreds of conditions, including temperature, humidity, wind speeds, altitude, and gunpowder varieties.
Creating a table for just one category of shell shot by one gun might require calculating three thousand trajectories from a set of differential equations. The work was often done using one of the Differential Analyzers invented at MIT by Vannevar Bush. The machine’s calculations were combined with the labor of more than 170 people, most of them women, known as “computers,” who tackled equations by punching the keys and cranking the handles of desktop adding machines. Women math majors were recruited from around the nation. But even with all of this effort, it took more than a month to complete just one firing table. By the summer of 1942, it was clear that production was falling further behind every week, rendering some of America’s artillery ineffective.
That August, Mauchly wrote a memo that proposed a way to help the Army meet this challenge. It would change the course of computing. Titled “The Use of High Speed Vacuum Tube Devices for Calculating,” his memo requested funding for the machine that he and Eckert were hoping to build: a digital electronic computer, using circuits with vacuum tubes, that could solve differential equations and perform other mathematical tasks. “A great gain in the speed of calculation can be obtained if the devices which are used employ electronic means,” he argued. He went on to estimate that a missile trajectory could be calculated in “100 seconds.”66
Mauchly’s memo was ignored by Penn’s deans, but it was brought to the attention of the Army officer attached to the university, Lieutenant (soon to be Captain) Herman Goldstine, a twenty-nine-year-old who had been a math professor at the University of Michigan. His mission was to speed up the production of firing tables, and he had dispatched his wife, Adele, also a mathematician, on a cross-country tour to recruit more women to join the battalions of human computers at Penn. Mauchly’s memo convinced him that there was a better way.
The decision of the U.S. War Department to fund the electronic computer came on April 9, 1943. Mauchly and Eckert stayed up all the night before working on their proposal, but they still hadn’t finished it by the time they got into the car for the two-hour ride from Penn to the Aberdeen Proving Ground in Maryland, where officials from the Ordnance Department were gathered. As Lieutenant Goldstine drove, they sat in the backseat writing the remaining sections, and when they arrived in Aberdeen, they continued working in a small room while Goldstine went to the review meeting. It was chaired by Oswald Veblen, the president of the Institute for Advanced Study in Princeton, who was advising the military on mathematical projects. Also present was Colonel Leslie Simon, director of the Army’s Ballistic Research Laboratory. Goldstine recalled what happened: “Veblen, after listening for a short while to my presentation and teetering on the back legs of his chair, brought the chair down with a crash, arose, and said, ‘Simon, give Goldstine the money.’ He thereupon left the room and the meeting ended on this happy note.”67
Mauchly and Eckert incorporated their memo into a paper they titled “Report on an Electronic Diff. Analyzer.” Using the abbreviation diff. was cagey; it stood for both differences, which reflected the digital nature of the proposed machine, and differential, which described the equations it would tackle. Soon it was given a more memorable name: ENIAC, the Electronic Numerical Integrator and Computer. Even though ENIAC was designed primarily for handling differential equations, which were key to calculating missile trajectories, Mauchly wrote that it could have a “programming device” that would allow it to do other tasks, thus making it more of a general-purpose computer.68
In June 1943 construction of ENIAC began. Mauchly, who retained his teaching duties, served as a consultant and visionary. Goldstine, as the Army’s representative, oversaw the operations and budget. And Eckert, with his passion for detail and perfection, was the chief engineer. Eckert became so dedicated to the project that he would sometimes sleep next to the machine. Once, as a joke, two engineers picked up his cot and gently moved him to an identical room one floor up; when he awoke he briefly feared the machine had been stolen.69
Knowing that great conceptions are worth little without precision execution (a lesson Atanasoff learned), Eckert was not shy about micromanaging. He would hover over the other engineers and tell them where to solder a joint or twist a wire. “I took every engineer’s work and checked every calculation of every resistor in the machine to make sure that it was done correctly,” he asserted. He disdained anyone who dismissed an issue as trivial. “Life is made up of a whole concentration of trivial matters,” he once said. “Certainly a computer is nothing but a huge concentration of trivial matters.”70
Eckert and Mauchly served as counterbalances for each other, which made them typical of so many digital-age leadership duos. Eckert drove people with a passion for precision; Mauchly tended to calm them and make them feel loved. “He was always kidding and joking with people,” Eckert recalled. “He was personable.” Eckert, whose technical skills came with a nervous energy and scattershot attention span, badly needed an intellectual sounding board, and Mauchly loved being that. Although he was not an engineer, Mauchly did have the ability to connect scientific theories with engineering practicalities in a way that was inspiring. “We got together and did this thing and I don’t think either of us would have done it by ourselves,” Eckert later conceded.71
ENIAC was digital, but instead of a binary system, using just 0s and 1s, it used a decimal system of ten-digit counters. In that regard, it was not like a modern computer. Other than that, it was more advanced than the machines built by Atanasoff, Zuse, Aiken, and Stibitz. Using what was called conditional branching (a capability described by Ada Lovelace a century earlier), it could hop around in a program based on its interim results, and it could repeat blocks of code, known as subroutines, that performed common tasks. “We had the ability to have subroutines and subroutines of subroutines,” Eckert explained. When Mauchly proposed this functionality, Eckert recalled, “it was an idea that I instantly recognized as the key to this whole thing.”72
After a year of building, around the time of D-Day in June 1944, Mauchly and Eckert were able to test the first two components, amounting to about one-sixth of the planned machine. They started with a simple multiplication problem. When it produced the correct answer, they let out a shout. But it took more than another year, until November 1945, for ENIAC to be fully operational. At that point it was able to perform five thousand additions and subtractions in one second, which was more than a hundred times faster than any previous machine. A hundred feet long and eight feet high, filling the space of what could be a modest three-bedroom apartment, it weighed close to thirty tons and had 17,468 vacuum tubes. By contrast, the Atanasoff-Berry computer, then languishing in a basement in Iowa, was the size of a desk, had only three hundred tubes, and could do merely thirty additions or subtractions per second.
BLETCHLEY PARK
Although few outsiders knew it at the time—and would not know for more than three decades—another electronic computer using vacuum tubes had been secretly built at the end of 1943 on the grounds of a redbrick Victorian manor in the town of Bletchley, fifty-four miles northwest of London, where the British had sequestered a team of geniuses and engineers to break the German wartime codes. The computer, known as Colossus, was the first all-electronic, partially programmable computer. Because it was geared for a special task, it was not a general-purpose or “Turing-complete” computer, but it did have Alan Turing’s personal fingerprints on it.
Turing had begun to focus on codes and cryptology in the fall of 1936, when he arrived at Princeton just after writing “On Computable Numbers.” He explained his interest in a letter to his mother that October:
I have just discovered a possible application of the kind of thing I am working on at present. It answers the question “What is the most general kind of code or cipher possible,” and at the same time (rather naturally) enables me to construct a lot of particular and interesting codes. One of them is pretty well impossible to decode without the key, and very quick to encode. I expect I could sell them to H.M. Government for quite a substantial sum, but am rather doubtful about the morality of such things. What do you think?73
Over the ensuing year, as he worried about the possibility of war with Germany, Turing got more interested in cryptology and less interested in trying to make money from it. Working in the machine shop of Princeton’s physics building in late 1937, he constructed the first stages of a coding machine that turned letters into binary numbers and, using electromechanical relay switches, multiplied the resulting numerically encoded message by a huge secret number, making it almost impossible to decrypt.
One of Turing’s mentors in Princeton was John von Neumann, the brilliant physicist and mathematician who had fled his native Hungary and was at the Institute for Advanced Study, which for the time being was located in the building that housed the university’s Mathematics Department. In the spring of 1938, as Turing was finishing his doctoral thesis, von Neumann offered him a job as his assistant. With the war clouds gathering in Europe, the offer was tempting, but it also felt vaguely unpatriotic. Turing decided to return to his fellowship at Cambridge and shortly thereafter joined the British effort to crack the German military codes.
His Majesty’s Government Code and Cypher School was, at the time, located in London and staffed mainly by literary scholars, such as Dillwyn “Dilly” Knox, a classics professor from Cambridge, and Oliver Strachey, a dilettante socialite who played piano and occasionally wrote about India. There were no mathematicians among the eighty staffers until the fall of 1938, when Turing went there. But the following summer, as Britain prepared for war, the department began actively hiring mathematicians, at one point using a contest that involved solving the Daily Telegraph crossword puzzle as a recruitment tool, and it relocated to the drab redbrick town of Bletchley, whose main distinction was being at the juncture where the railway line between Oxford and Cambridge intersected with the one from London to Birmingham. A team from the British intelligence service, posing as “Captain Ridley’s shooting party,” visited the Bletchley Park manor house, a Victorian Gothic monstrosity that its owner wanted to demolish, and discreetly bought it. The code breakers were located in the cottages, stables, and some prefabricated huts that were erected on the grounds.74
Turing was assigned to a team working in Hut 8 that was trying to break the German Enigma code, which was generated by a portable machine with mechanical rotors and electrical circuits. It encrypted military messages by using a cipher that, after every keystroke, changed the formula for substituting letters. That made it so tough to decipher that the British despaired of ever doing so. A break came when Polish intelligence officers created a machine based on a captured German coder that was able to crack some of the Enigma codes. By the time the Poles showed the British their machine, however, it had been rendered ineffective because the Germans had added two more rotors and two more plugboard connections to their Enigma machines.
Turing and his team went to work creating a more sophisticated machine, dubbed “the bombe,” that could decipher the improved Enigma messages—in particular, naval orders that would reveal the deployment of U-boats that were decimating British supply convoys. The bombe exploited a variety of subtle weaknesses in the coding, including the fact that no letter could be enciphered as itself and that there were certain phrases the Germans used repeatedly. By August 1940 Turing’s team had two operating bombes, which were able to break 178 coded messages; by the end of the war they had built close to two hundred.
The Turing-designed bombe was not a notable advance in computer technology. It was an electromechanical device with relay switches and rotors rather than vacuum tubes and electronic circuits. But a subsequent machine produced at Bletchley Park, and Colossus, was a major milestone.
The need for Colossus arose when the Germans started coding important messages, such as orders from Hitler and his high command, with an electronic digital machine that used a binary system and twelve code wheels of unequal size. The electromechanical bombes designed by Turing were powerless to break it. It required an attack using lightning-quick electronic circuits.
The team in charge, based in Hut 11, was known as the Newmanry after its leader, Max Newman, the Cambridge math don who had introduced Turing to Hilbert’s problems almost a decade earlier. Newman’s engineering partner was the electronics wizard Tommy Flowers, a pioneer of vacuum tubes, who worked at the Post Office Research Station at Dollis Hill, a London suburb.
Turing was not part of Newman’s team, but he did come up with a statistical approach, dubbed “Turingery,” that detected any departures from a uniform distribution of characters in a stream of ciphered text. A machine was built that could scan two loops of punched paper tapes, using photoelectric heads, in order to compare all possible permutations of the two sequences. The machine was dubbed the “Heath Robinson,” after a British cartoonist who specialized, as did Rube Goldberg in America, in drawing absurdly complex mechanical contraptions.
For almost a decade Flowers had been fascinated by electronic circuits made with vacuum tubes, which he and other Brits called “valves.” As an engineer with the Post Office’s telephone division, he had created in 1934 an experimental system that used more than three thousand tubes to control connections among a thousand phone lines. He also pioneered the use of vacuum tubes for data storage. Turing enlisted Flowers to help on the bombe machines and then introduced him to Newman.
Flowers realized that the only way to analyze the German encrypted streams quickly enough was to store at least one of them into the internal electronic memory of a machine rather than trying to compare two punched paper tapes. This would require 1,500 vacuum tubes. At first the Bletchley Park managers were skeptical, but Flowers pushed ahead, and by December 1943—after only eleven months—he produced the first Colossus machine. An even bigger version, using 2,400 vacuum tubes, was ready by June 1, 1944. Its first decoded intercepts supported other sources informing General Dwight Eisenhower, who was about to launch the D-Day invasion, that Hitler was not ordering extra troops to Normandy. Within a year, eight more Colossus machines were produced.
This meant that well before ENIAC, which did not become operational until November 1945, the British code breakers had built a fully electronic and digital (indeed binary) computer. The second version, in June 1944, was even capable of some conditional branching. But unlike ENIAC, which had ten times the number of tubes, Colossus was a special-purpose machine geared for code breaking, not a general-purpose computer. With its limited programmability, it could not be instructed to perform all computational tasks, the way that (in theory) ENIAC could.
SO, WHO INVENTED THE COMPUTER?
In assessing how to apportion credit for creating the computer, it’s useful to begin by specifying which attributes define the essence of a computer. In the most general sense, the definition of a computer could encompass everything from an abacus to an iPhone. But in chronicling the birth of the Digital Revolution, it makes sense to follow the accepted definitions of what, in modern usage, constitutes a computer. Here are a few:
“A programmable usually electronic device that can store, retrieve, and process data.” (Merriam-Webster Dictionary)
“An electronic device which is capable of receiving information (data) in a particular form and of performing a sequence of operations in accordance with a predetermined but variable set of procedural instructions (program) to produce a result.” (Oxford English Dictionary)
“A general purpose device that can be programmed to carry out a set of arithmetic or logical operations automatically.” (Wikipedia, 2014)
So the ideal computer is a machine that is electronic, general purpose, and programmable. What, then, best qualifies as the first?
George Stibitz’s Model K, begun on his kitchen table in November 1937, led to a full-scale model at Bell Labs in January 1940. It was a binary computer and the first such device to be used remotely. But it used electromechanical relays and was thus not fully electronic. It was also a special-purpose computer and not programmable.
Herman Zuse’s Z3, completed in May 1941, was the first automatically controlled, programmable, electrical, binary machine. It was designed to do engineering problems rather than be a general-purpose machine. However, it was later shown that, in theory, it could have been used as a Turing-complete machine. Its major difference from modern computers was that it was electromechanical, dependent on clacking and slow relay switches, rather than electronic. Another shortcoming is that it never really went into full-scale service. It was destroyed by the Allied bombing of Berlin in 1943.
The computer designed by John Vincent Atanasoff, which was complete but not fully workable by the time Atanasoff abandoned it to serve in the Navy in September 1942, was the world’s first electronic digital computer, but it was only partly electronic. Its add-subtract mechanism used vacuum tubes, but its memory and data retrieval involved mechanical rotating drums. Its other main drawback, in terms of being considered the first modern computer, was that it was not programmable nor general purpose; instead it was hard-wired for the special task of solving linear equations. Also, Atanasoff never got it fully operational, and it disappeared into a basement at Iowa State.
Bletchley Park’s Colossus I, completed in December 1943 by Max Newman and Tommy Flowers (with input from Alan Turing), was the first digital computer that was fully electronic, programmable, and operational. It was not, however, a general-purpose or Turing-complete machine; it was geared to the specific purpose of breaking Germany’s wartime codes.
Howard Aiken’s Harvard Mark I, built with IBM and put into operation in May 1944, was programmable, as we will see in the following chapter, but it was electromechanical rather than electronic.
ENIAC, completed by Presper Eckert and John Mauchly in November 1945, was the first machine to incorporate the full set of traits of a modern computer. It was all-electronic, superfast, and could be programmed by plugging and unplugging the cables connecting its different units. It was capable of changing paths based on interim results, and it qualified as a general-purpose Turing-complete machine, meaning it could in theory tackle any task. Most important, it worked. “That’s a big thing with an invention,” Eckert later said, contrasting their machine with Atanasoff’s. “You have to have a whole system that works.”75 Mauchly and Eckert got their machine to do some very powerful calculations, and it was in constant use for ten years. It became the basis for most subsequent computers.
That last attribute is important. When we ascribe credit for an invention, determining who should be most noted by history, one criterion is looking at whose contributions turned out to have the most influence. Invention implies contributing something to the flow of history and affecting how an innovation developed. Using historic impact as a standard, Eckert and Mauchly are the most noteworthy innovators. Almost all computers of the 1950s trace their roots to ENIAC. The influence of Flowers, Newman, and Turing is somewhat trickier to assess. Their work was kept top-secret, but all three men were involved in the British computers built after the war. Zuse, who was isolated and under bombardment in Berlin, had even less influence on the course of computer development elsewhere. As for Atanasoff, his main influence on the field, perhaps his only influence, came from providing a few inspirations to Mauchly when he visited.
The issue of what inspirations Mauchly gleaned during his four-day visit with Atanasoff in Iowa in June 1941 turned into a protracted legal dispute. That raised another criterion, more legalistic than historical, in assessing credit for invention: Who, if anyone, ended up with the patents? In the case of the first computers, nobody did. But that outcome was due to a controversial legal battle that resulted in the patents of Eckert and Mauchly being nullified.76
The saga began in 1947, when Eckert and Mauchly, after leaving Penn, applied for a patent on their ENIAC work, which was finally granted (the patent system being rather slow) in 1964. By then the Eckert-Mauchly company and its patent rights had been sold to Remington Rand, which became Sperry Rand; it began pressuring other companies to pay it licensing fees. IBM and Bell Labs made deals, but Honeywell balked and started looking for a way to challenge the patents. It hired a young lawyer, Charles Call, who had an engineering degree and had worked at Bell Labs. His mission was to upend the Eckert-Mauchly patent by showing that their ideas weren’t original.
Pursuing a tip from a Honeywell lawyer who had gone to Iowa State and read about the computer that Atanasoff had built there, Call paid a visit to Atanasoff at his home in Maryland. Atanasoff was charmed by Call’s knowledge of his computer and somewhat resentful that he had never gotten much credit for it, so he handed over hundreds of letters and documents that showed how Mauchly had derived some ideas from his visit to Iowa. That evening Call drove to Washington to sit in the back of a lecture Mauchly was giving. In answer to a question about Atanasoff’s machine, Mauchly claimed he had barely examined it. Call realized that if he could get Mauchly to say this in a deposition, then he could discredit him at a trial by producing Atanasoff’s documents.
When Mauchly found out a few months later that Atanasoff might be helping Honeywell challenge his patents, he made his own visit to Atanasoff’s Maryland home, bringing with him a Sperry Rand lawyer. It was an awkward meeting. Mauchly claimed that during his visit to Iowa he hadn’t read Atanasoff’s paper carefully or examined his computer, and Atanasoff coldly pointed out that this was not true. Mauchly stayed for dinner and tried to ingratiate himself with Atanasoff, but to no avail.
The issue went to trial before a federal judge, Earl Larson, in Minneapolis in June 1971. Mauchly proved a problematic witness. Pleading poor memory, he sounded squirrely about what he had seen during his visit to Iowa, and he repeatedly backtracked from assertions he had made in his earlier deposition, including his claim that he had only seen Atanasoff’s computer partly covered and in dim light. Atanasoff, by contrast, was very effective. He described the machine he had built, demonstrated a model, and pointed out which of his ideas Mauchly had borrowed. In all, seventy-seven witnesses were called to testify, another eighty were deposed, and 32,600 exhibits were entered into the record. The trial lasted more than nine months, making it the longest federal trial to that point.
Judge Larson took another nineteen months to write his final decision, which was issued in October 1973. In it he ruled that the Eckert-Mauchly ENIAC patent was invalid: “Eckert and Mauchly did not themselves first invent the automatic electronic digital computer, but instead derived that subject matter from one Dr. John Vincent Atanasoff.”77 Instead of appealing, Sperry settled with Honeywell.IV
The judge’s opinion, at 248 pages, was thorough, but it disregarded some significant differences between the machines. Mauchly did not derive quite as much from Atanasoff as the judge seemed to think. For example, Atanasoff’s electronic circuit used binary logic, whereas Mauchly’s was a decimal counter. Had the Eckert-Mauchly patent claims been less sweeping, they probably would have survived.