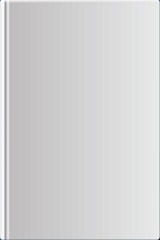
Текст книги "Iptables Tutorial 1.2.2"
Автор книги: Oskar Andreasson
Жанр:
Интернет
сообщить о нарушении
Текущая страница: 2 (всего у книги 30 страниц)
IP characteristics
The IP protocol resides in the Internet layer, as we have already said. The IP protocol is the protocol in the TCP/IP stack that is responsible for letting your machine, routers, switches and etcetera, know where a specific packet is going. This protocol is the very heart of the whole TCP/IP stack, and makes up the very foundation of everything in the Internet.
The IP protocol encapsulates the Transport layer packet with information about which Transport layer protocol it came from, what host it is going to, and where it came from, and a little bit of other useful information. All of this is, of course, extremely precisely standardized, down to every single bit. The same applies to every single protocol that we will discuss in this chapter.
The IP protocol has a couple of basic functionalities that it must be able to handle. It must be able to define the datagram, which is the next building block created by the transport layer (this may in other words be TCP, UDP or ICMP for example). The IP protocol also defines the Internet addressing system that we use today. This means that the IP protocol is what defines how to reach between hosts, and this also affects how we are able to route packets, of course. The addresses we are talking about are what we generally call an IP address. Usually when we talk about IP addresses, we talk about dotted quad numbers (e.g., 127.0.0.1). This is mostly to make the IP addresses more readable for the human eye, since the IP address is actually just a 32 bit field of 1's and 0's (127.0.0.1 would hence be read as 01111111000000000000000000000001 within the actual IP header).
The IP protocol has even more magic it must perform up it's sleeve. It must also be able to decapsulate and encapsulate the IP datagram (IP data) and send or receive the datagram from either the Network access layer, or the transport layer. This may seem obvious, but sometimes it is not. On top of all this, it has two big functions it must perform as well, that will be of quite interest for the firewalling and routing community. The IP protocol is responsible for routing packets from one host to another, as well as packets that we may receive from one host destined for another. Most of the time on single network access host, this is a very simple process. You have two different options, either the packet is destined for our locally attached network, or possibly through a default gateway. but once you start working with firewalls or security policies together with multiple network interfaces and different routes, it may cause quite some headache for many network administrators. The last of the responsibilities for the IP protocol is that it must fragment and reassemble any datagram that has previously been fragmented, or that needs to be fragmented to fit in to the packetsize of this specific network hardware topology that we are connected to. If these packet fragments are sufficiently small, they may cause a horribly annoying headache for firewall administrators as well. The problem is, that once they are fragmented to small enough chunks, we will start having problems to read even the headers of the packet, not to mention the actual data.
Tip As of Linux kernel 2.4 series, and iptables, this should no longer be a problem for most linux firewalls. The connection tracking system used by iptables for state matching and NAT'ing etc must be able to read the packet defragmented. Because of this, conntrack automatically defragments all packets before they reach the netfilter/iptables structure in the kernel.
The IP protocol is also a connectionless protocol, which in turn means that IP does not "negotiate" a connection. a connection-oriented protocol on the other hand negotiates a connection (called a handshake) and then when all data has been sent, tears it down. TCP is an example of this kind of protocol, however, it is implemented on top of the IP protocol. The reason for not being connection-oriented just yet are several, but among others, a handshake is not required at this time yet since there are other protocols that this would add an unnecessarily high overhead to, and that is made up in such a way that if we don't get a reply, we know the packet was lost somewhere in transit anyways, and resend the original request. As you can see, sending the request and then waiting for a specified amount of time for the reply in this case, is much preferred over first sending one packet to say that we want to open a connection, then receive a packet letting us know it was opened, and finally acknowledge that we know that the whole connection is actually open, and then actually send the request, and after that send another packet to tear the connection down and wait for another reply.
IP is also known as an unreliable protocol, or simply put it does not know if a packet was received or not. It simply receives a packet from the transport layer and does its thing, and then passes it on to the network access layer, and then nothing more to it. It may receive a return packet, which traverses from network access layer to the IP protocol which does it's thing again, and then passes it on upwards to the Transport layer. However, it doesn't care if it gets a reply packet, or if the packet was received at the other end. Same thing applies for the unreliability of IP as for the connectionless-ness, since unreliability would require adding an extra reply packet to each packet that is sent. For example, let us consider a DNS lookup. As it is, we send a DNS request for servername.com. If we never receive a reply, we know something went wrong and re-request the lookup, but during normal use we would send out one request, and get one reply back. Adding reliability to this protocol would mean that the request would require two packets (one request, and one confirmation that the packet was received) and then two packets for the reply (one reply, and one reply to acknowledge the reply was received). In other words, we just doubled the amount of packets needed to send, and almost doubled the amount of data needed to be transmitted.
IP headers
The IP packet contains several different parts in the header as you have understood from the previous introduction to the IP protocol. The whole header is meticuluously divided into different parts, and each part of the header is allocated as small of a piece as possible to do it's work, just to give the protocol as little overhead as possible. You will see the exact configuration of the IP headers in the IP headers image.
Note Understand that the explanations of the different headers are very brief and that we will only discuss the absolute basics of them. For each type of header that we discuss, we will also list the proper RFC's that you should read for further understanding and technical explanations of the protocol in question. As a sidenote to this note, RFC stands for Request For Comments, but these days, they have a totally different meaning to the Internet community. They are what defines and standardises the whole Internet, compared to what they were when the researchers started writing RFC's to each other. Back then, they were simply requests for comments and a way of asking other researchers about their opinions.
The IP protocol is mainly described in RFC 791 – Internet Protocol. However, this RFC is also updated by RFC 1349 – Type of Service in the Internet Protocol Suite, which was obsoleted by RFC 2474 – Definition of the Differentiated Services Field (DS Field) in the IPv4 and IPv6 Headers, and which was updated by RFC 3168 – The Addition of Explicit Congestion Notification (ECN) to IP and RFC 3260 – New Terminology and Clarifications for Diffserv.
Tip As you can see, all of these standards can get a little bit hard to follow at times. One tip for finding the different RFC's that are related to each other is to use the search functions available at RFC-editor.org. In the case of IP, consider that the RFC 791 is the basic RFC, and all of the other are simply updates and changes to that standard. We will discuss these more in detail when we get to the specific headers that are changed by these newer RFC's.
One thing to remember is, that sometimes, an RFC can be obsoleted (not used at all). Normally this means that the RFC has been so drastically updated and that it is better to simply replace the whole thing. It may also become obsolete for other reasons as well. When an RFC becomes obsoleted, a field is added to the original RFC that points to the new RFC instead.

Version – bits 0-3. This is a version number of the IP protocol in binary. IPv4 iscalled 0100, while IPv6 is called 0110. This field is generally not used for filtering very much. The version described in RFC 791 is IPv4.
IHL (Internet Header Length) – bits 4-7. This field tells us how long the IP header is in 32 bit words. As you can see, we have split the header up in this way (32 bits per line) in the image as well. Since the Options field is of optional length, we can never be absolutely sure of how long the whole header is, without this field. The minimum length of this of the header is 5 words.
Type of Service, DSCP, ECN – bits 8-15. This is one of the most complex areas of the IP header for the simple reason that it has been updated 3 times. It has always had the same basic usage, but the implementation has changed several times. First the field was called the Type of Service field. Bit [0-2] of the field was called the Precedence field. Bit [3] was Normal/Low delay, Bit [4] was Normal/High throughput, Bit [5] was Normal/High reliability and bit [6-7] was reserved for future usage. This is still used in a lot of places with older hardware, and it still causes some problems for the Internet. Among other things, bit [6-7] are specified to be set to 0. In the ECN updates (RFC 3168, we start using these reserved bits and hence set other values than 0 to these bits. But a lot of old firewalls and routers have built in checks looking if these bits are set to 1, and if the packets do, the packet is discarded. Today, this is clearly a violation of RFC's, but there is not much you can do about it, except to complain.
The second iteration of this field was when the field was changed into the DS field as defined in RFC 2474. DS stands for Differentiated Services. According to this standard bits [0-5] is Differentiated Services Code Point (DSCP) and the remaining two bits [6-7] are still unused. The DSCP field is pretty much used the same as in how the ToS field was used before, to mark what kind of service this packet should be treated like if the router in question makes any difference between them. One big change is that a device must ignore the unused bits to be fully RFC 2474 compliant, which means we get rid of the previous hassle as explained previously, as long as the device creators follow this RFC.
The third, and almost last, change of the ToS field was when the two, previously, unused bits were used for ECN (Explicit Congestion Notification), as defined in RFC 3168. ECN is used to let the end nodes know about a routers congestion, before it actually starts dropping packets, so that the end nodes will be able to slow down their data transmissions, before the router actually needs to start dropping data. Previously, dropping data was the only way that a router had to tell that it was overloaded, and the end nodes had to do a slow restart for each dropped packet, and then slowly gather up speed again. The two bits are named ECT (ECN Capable Transport) and CE (Congestion Experienced) codepoints.
The final iteration of the whole mess is RFC 3260 which gives some new terminology and clarifications to the usage of the DiffServ system. It doesn't involve too many new updates or changes, except in the terminology. The RFC is also used to clarify some points that were discussed between developers.
Total Length – bits 16 – 31. This field tells us how large the packet is in octets, including headers and everything. The maximum size is 65535 octets, or bytes, for a single packet. The minimum packet size is 576 bytes, not caring if the packet arrives in fragments or not. It is only recommended to send larger packets than this limit if it can be guaranteed that the host can receive it, according to RFC 791. However, these days most networks runs at 1500 byte packet size. This includes almost all ethernet connections, and most Internet connections.
Identification – bits 32 – 46. This field is used in aiding the reassembly of fragmented packets.
Flags – bits 47 – 49. This field contains a few miscellaneous flags pertaining to fragmentation. The first bit is reserved, but still not used, and must be set to 0. The second bit is set to 0 if the packet may be fragmented, and to 1 if it may not be fragmented. The third and last bit can be set to 0 if this was the last fragment, and 1 if there are more fragments of this same packet.
Fragment Offset – bits 50 – 63. The fragment offset field shows where in the datagram that this packet belongs. The fragments are calculated in 64 bits, and the first fragment has offset zero.
Time to live – bits 64 – 72. The TTL field tells us how long the packet may live, or rather how many "hops" it may take over the Internet. Every process that touches the packet must remove one point from the TTL field, and if the TTL reaches zero, the whole packet must be destroyed and discarded. This is basically used as a safety trigger so that a packet may not end up in an uncontrollable loop between one or several hosts. Upon destruction the host should return an ICMP Time exceeded message to the sender.
Protocol – bits 73 – 80. In this field the protocol of the next level layer is indicated. For example, this may be TCP, UDP or ICMP among others. All of these numbers are defined by the Internet Assigned Numbers Authority. All numbers can befound on their homepage Internet Assigned Numbers Authority.
Header checksum – bits 81 – 96. This is a checksum of the IP header of the packet.This field is recomputed at every host that changes the header, which means pretty much every host that the packet traverses over, since they most often change the packets TTL field or some other.
Source address – bits 97 – 128. This is the source address field. It is generally written in 4 octets, translated from binary to decimal numbers with dots in between. That is for example, 127.0.0.1. The field lets the receiver know where the packet came from.
Destination address – bits 129 – 160. The destination address field contains the destination address, and what a surprise, it is formatted the same way as the source address.
Options – bits 161 – 192 <> 478. The options field is not optional, as it may sound. Actually, this is one of the more complex fields in the IP header. The options field contains different optional settings within the header, such as Internet timestamps, SACK or record route route options. Since these options are all optional, the Options field can have different lengths, and hence the whole IP header. However, since we always calculate the IP header in 32 bit words, we must always end the header on an even number, that is the multiple of 32. The field may contain zero or more options.
The options field starts with a brief 8 bit field that lets us know which options are used in the packet. The options are all listed in the TCP Options table, in the TCP options appendix. For more information about the different options, read the proper RFC's. For an updated listing of the IP options, check at Internet Assigned Numbers Authority.
Padding – bits variable. This is a padding field that is used to make the header end at an even 32 bit boundary. The field must always be set to zeroes straight through to the end.
TCP characteristics
The TCP protocol resides on top of the IP protocol. It is a stateful protocol and has built-in functions to see that the data was received properly by the other end host. The main goals of the TCP protocol is to see that data is reliably received and sent, that the data is transported between the Internet layer and Application layer correctly, and that the packet data reaches the proper program in the application layer, and that the data reaches the program in the right order. All of this is possible through the TCP headers of the packet.
The TCP protocol looks at data as an continuous data stream with a start and a stop signal. The signal that indicates that a new stream is waiting to be opened is called a SYN three-way handshake in TCP, and consists of one packet sent with the SYN bit set. The other end then either answers with SYN/ACK or SYN/RST to let the client know if the connection was accepted or denied, respectively. If the client receives an SYN/ACK packet, it once again replies, this time with an ACK packet. At this point, the whole connection is established and data can be sent. During this initial handshake, all of the specific options that will be used throughout the rest of the TCP connection is also negotiated, such as ECN, SACK, etcetera.
While the datastream is alive, we have further mechanisms to see that the packets are actually received properly by the other end. This is the reliability part of TCP. This is done in a simple way, using a Sequence number in the packet. Every time we send a packet, we give a new value to the Sequence number, and when the other end receives the packet, it sends an ACK packet back to the data sender. The ACK packet acknowledges that the packet was received properly. The sequence number also sees to it that the packet is inserted into the data stream in a good order.
Once the connection is closed, this is done by sending a FIN packet from either end-point. The other end then responds by sending a FIN/ACK packet. The FIN sending end can then no longer send any data, but the other end-point can still finish sending data. Once the second end-point wishes to close the connection totally, it sends a FIN packet back to the originally closing end-point, and the other end-point replies with a FIN/ACK packet. Once this whole procedure is done, the connection is torn down properly.
As you will also later see, the TCP headers contain a checksum as well. The checksum consists of a simple hash of the packet. With this hash, we can with rather high accuracy see if a packet has been corrupted in any way during transit between the hosts.
TCP headers
The TCP headers must be able to perform all of the tasks above. We have already explained when and where some of the headers are used, but there are still other areas that we haven't touched very deeply at. Below you see an image of the complete set of TCP headers. It is formatted in 32 bit words per row, as you can see.

Source port – bit 0 – 15. This is the source port of the packet. The source port was originally bound directly to a process on the sending system. Today, we use a hash between the IP addresses, and both the destination and source ports to achieve this uniqueness that we can bind to a single application or program.
Destination port – bit 16 – 31. This is the destination port of the TCP packet. Just as with the source port, this was originally bound directly to a process on the receiving system. Today, a hash is used instead, which allows us to have more open connections at the same time. When a packet is received, the destination and source ports are reversed in the reply back to the originally sending host, so that destination port is now source port, and source port is destination port.
Sequence Number – bit 32 – 63. The sequence number field is used to set a number on each TCP packet so that the TCP stream can be properly sequenced (e.g., the packets winds up in the correct order). The Sequence number is then returned in the ACK field to ackonowledge that the packet was properly received.
Acknowledgment Number – bit 64 – 95. This field is used when we acknowledge a specific packet a host has received. For example, we receive a packet with one Sequence number set, and if everything is okey with the packet, we reply with an ACK packet with the Acknowledgment number set to the same as the original Sequence number.
Data Offset – bit 96 – 99. This field indicates how long the TCP header is, and where the Data part of the packet actually starts. It is set with 4 bits, and measures the TCP header in 32 bit words. The header should always end at an even 32 bit boundary, even with different options set. This is possible thanks to the Padding field at the very end of the TCP header.
Reserved – bit 100 – 103. These bits are reserved for future usage. In RFC 793 this also included the CWR and ECE bits. According to RFC 793 bit 100-105 (i.e., this and the CWR and ECE fields) must be set to zero to be fully compliant. Later on, when we started introducing ECN, this caused a lot of troubles because a lot of Internet appliances such as firewalls and routers dropped packets with them set. This is still true as of writing this.
CWR – bit 104. This bit was added in RFC 3268 and is used by ECN. CWR stands for Congestion Window Reduced, and is used by the data sending part to inform the receiving part that the congestion window has been reduced. When the congestion window is reduced, we send less data per timeunit, to be able to cope with the total network load.
ECE – bit 105. This bit was also added with RFC 3268 and is used by ECN. ECE stands for ECN Echo. It is used by the TCP/IP stack on the receiver host to let the sending host know that it has received an CE packet. The same thing applies here, as for the CWR bit, it was originally a part of the reserved field and because of this, some networking appliances will simply drop the packet if these fields contain anything else than zeroes. This is actually still true for a lot of appliances unfortunately.
URG – bit 106. This field tells us if we should use the Urgent Pointer field or not. If set to 0, do not use Urgent Pointer, if set to 1, do use Urgent pointer.
ACK – bit 107. This bit is set to a packet to indicate that this is in reply to another packet that we received, and that contained data. An Acknowledgment packet is always sent to indicate that we have actually received a packet, and that it contained no errors. If this bit is set, the original data sender will check the Acknowledgment Number to see which packet is actually acknowledged, and then dump it from the buffers.
PSH – bit 108. The PUSH flag is used to tell the TCP protocol on any intermediate hosts to send the data on to the actual user, including the TCP implementation on the receiving host. This will push all data through, unregardless of where or how much of the TCP Window that has been pushed through yet.
RST – bit 109. The RESET flag is set to tell the other end to tear down the TCP connection. This is done in a couple of different scenarios, the main reasons being that the connection has crashed for some reason, if the connection does not exist, or if the packet is wrong in some way.
SYN – bit 110. The SYN (or Synchronize sequence numbers) is used during the initial establishment of a connection. It is set in two instances of the connection, the initial packet that opens the connection, and the reply SYN/ACK packet. It should never be used outside of those instances.
FIN – bit 111. The FIN bit indicates that the host that sent the FIN bit has no more data to send. When the other end sees the FIN bit, it will reply with a FIN/ACK. Once this is done, the host that originally sent the FIN bit can no longer send any data. However, the other end can continue to send data until it is finished, and will then send a FIN packet back, and wait for the final FIN/ACK, after which the connection is sent to a CLOSED state.
Window – bit 112 – 127. The Window field is used by the receiving host to tell the sender how much data the receiver permits at the moment. This is done by sending an ACK back, which contains the Sequence number that we want to acknowledge, and the Window field then contains the maximum accepted sequence numbers that the sending host can use before he receives the next ACK packet. The next ACK packet will update accepted Window which the sender may use.
Checksum – bit 128 – 143. This field contains the checksum of the whole TCP header. It is a one's complement of the one's complement sum of each 16 bit word in the header. If the header does not end on a 16 bit boundary, the additional bits are set to zero. While the checksum is calculated, the checksum field is set to zero. The checksum also covers a 96 bit pseudoheader containing the Destination-, Source-address, protocol, and TCP length. This is for extra security.
Urgent Pointer – bit 144 – 159. This is a pointer that points to the end of the data which is considered urgent. If the connection has important data that should be processed as soon as possible by the receiving end, the sender can set the URG flag and set the Urgent pointer to indicate where the urgent data ends.
Options – bit 160 – **. The Options field is a variable length field and contains optional headers that we may want to use. Basically, this field contains 3 subfields at all times. An initial field tells us the length of the Options field, a second field tells us which options are used, and then we have the actual options. A complete listing of all the TCP Options can be found in TCP options.
Padding – bit **. The padding field pads the TCP header until the whole header ends at a 32-bit boundary. This ensures that the data part of the packet begins on a 32-bit boundary, and no data is lost in the packet. The padding always consists of only zeros.